Scientists estimate that the Sun was born 5 billion years ago, and the Universe starts about 13.4 billion years ago. Most of stars that we can see in the night sky were born millions or billions of years ago. Millions of years and billions of years are normal time scales in the Universe. Recent study in Astrophysical Journal Letter found[1] a remarkable neutron star, which is known as Swift J1818.0−1607: it is just 240 years old !
Discovery of Swift J1818.0−1607
On March 12th, 2020, the burst alert telescope on board Neil Gehrels Swift observatory (Swift for short) caught an intense outburst from the space outside the solar system[2]. Soon after, the Swift started a deep observation in the direction of the outburst, and found new X-ray source, which was namedSwift J1818.0−1607(Figure 1).Follow-up observations[3][4]confirmed that the radiation from Swift J1818.0−1607 is regularly blinking with a time interval 1.36 second and the time interval gets gradually longer and longer. Researchers eventually concluded that Swift J1818.0−1607 is a rapidly spinning and highly magnetized neutron star.
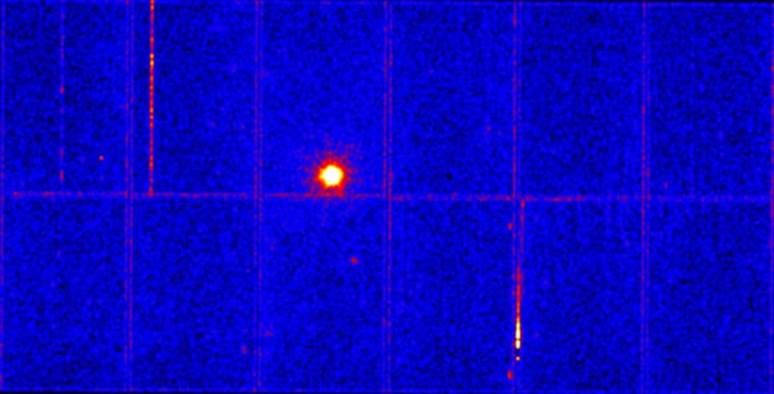
Figure 1:X-ray image of Swift J1818.0-1607, the youngest pulsar ever observed, as seen by the ESA’s XMM-Newton observatory. Image credit: ESA / XMM-Newton / P. Esposito et al.
What is the neutron star?
Neutron stars are one of the most exotic stars in the Universe. Its diameter is only about 20 km, which is typical size of the Earthly city (Figure 2), but its weight is about twice of our Sun, which is about half a million of the weight of the Earth. So, we can see that neutron stars are very dense. Neutron stars would be the second densest object in the Universe; just one cube centimeter of the neutron star matter weighs 100 trillion gram on Earth (the object with a higher density than a neutron star is a black hole). There is another thinking way how neutron star is so dense. We know that atoms are main building blocks of all materials and one atom is composed of a nucleus, which is made by the protons and neutrons making the nucleus, and electrons moving around the nucleus. One nucleus is about 100,000 times smaller than the atom; if the nucleus were size of a baseball, the atom would be about the size of the city. So, in terms of the atomic scale, usual matter is mostly consisting of empty space. Neutron star interior, however, is so dense that the separation between two nuclei is just size of one nucleus and normal atoms cannot exist anymore. Most of nuclei inside the neutron star dissolve to create a soup of free neutrons (this is the reason why this exotic star is called neutron star).
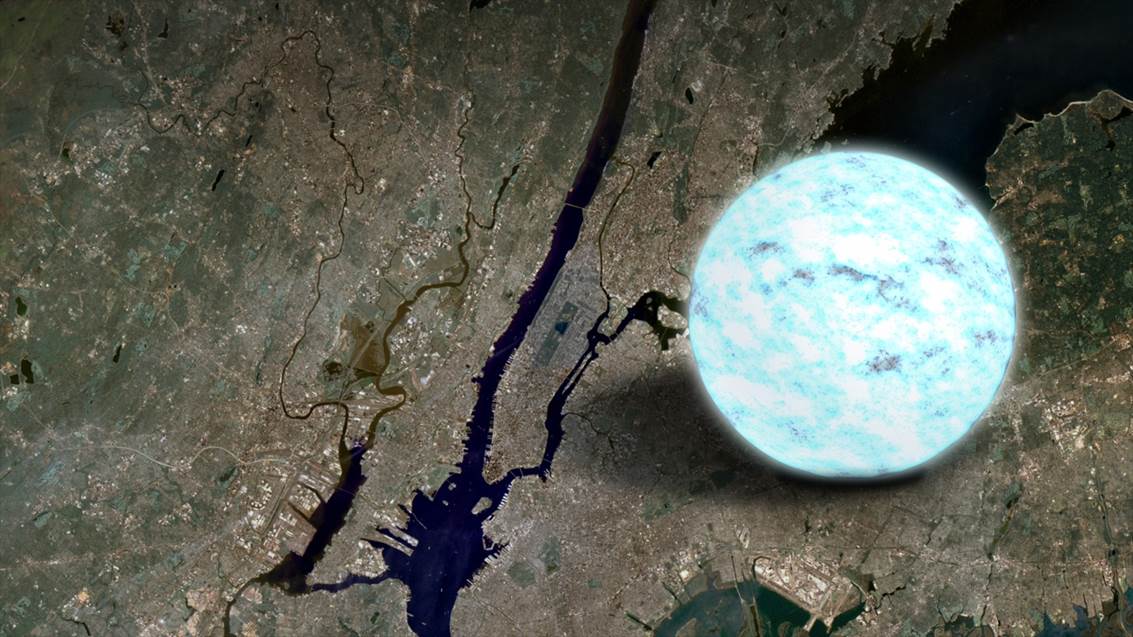
Figure 2:Neutron star is one of the densest objects, which is about 20 km across and half a million times Earth’s mass. Image credit:NASA's Goddard Space Flight Center
How neutron star is born? A normal star, such like our Sun, is stable due to the balance of the forces; the gravity force tries to compress the star, while the internal pressure pushes the star outward. The internal pressure is produced by the nuclear fusion at the center. This force balance suddenly comes to an end if the nuclear fuel is exhausted and the outward pressure is removed from the star. The gravity crushes the star and causes asupernova explosion, which blows the most matter of the star into space. This collapse leaves behind the dense and compact object, neutron star (Figure 3). Scientists consider that the main-sequence star with 8-30 solar mass produces a neutron star (a normal star with higher mass continues to collapse into a black hole). The first neutron star, which is known as PSR B1919+21 was discovered in 1967[5]. About 240 years ago, the light of the supernova explosion leaving Swift J1818.0−1607 reached on the Earth. People in Qing dynasty might catch the light from this explosion (note: Since the distance to Swift J1818.0−1607 is about 16,000 light-years away, the light observed in 240 years ago was emitted about 16,240 years ago).
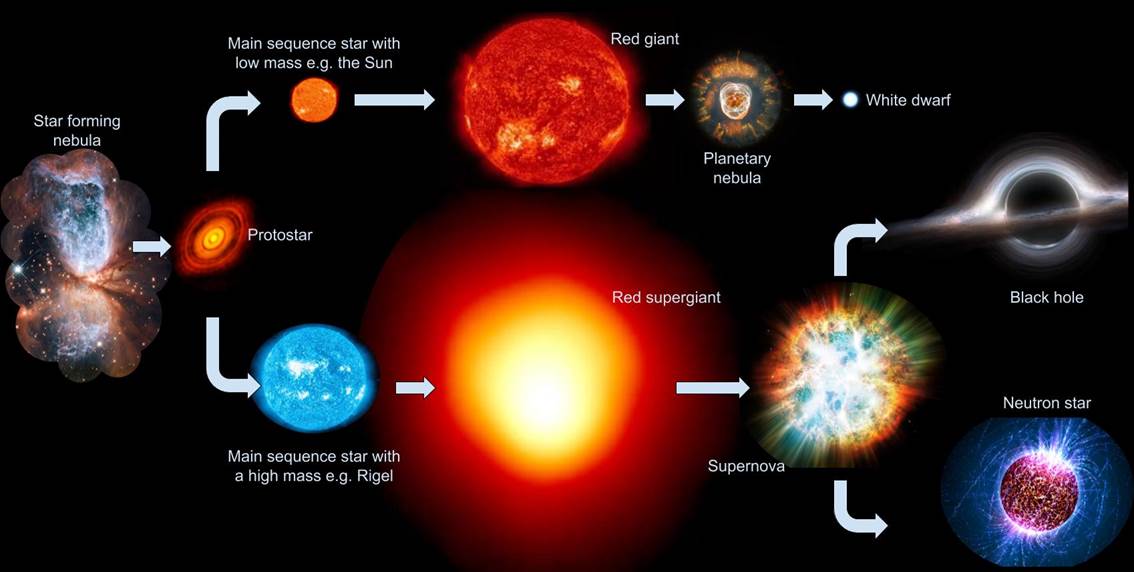
Figure 3: Life of the stars. Neutron star is born after supernova explosion of massive stars. Image credit: Mr Toogood's Physics http://www.alevelphysicsnotes.com/index.php
Spinning of neutron star
Spinning is a common property of the stars in the Universe; Earth spins once every 24 hours and Sun spins about once every 27 days. Astronomer found Swift J1818.0−1607 spins once every 1.36 seconds! Why do neutron stars spin so fast? The reason is similar to a spinning figure skater. We see that the figure skater spins faster when she pulls her arms to her body. This physic law is called angular momentum conservation: when a spinning object shrinks in size, the spinning speed increases with inversely proportional to the square of the arm length (Figure 4). For example, if the Sun, whose radius is about 0.7 billion meters, collapsed to the size of the neutron star, it would rotate once every 1 millisecond. So, neutron star can spin so fast.
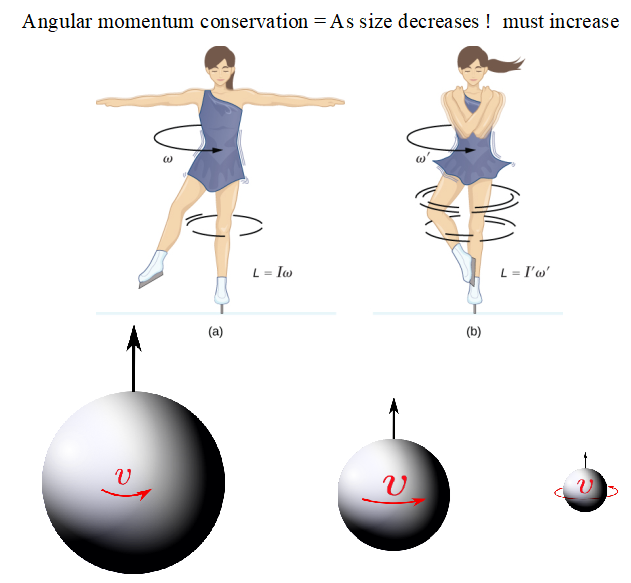
Figure 4: Angular momentum conservation. As spinning object shrinks in the size, the spinning speed increases. Image credit:© 1999-2020, Rice University, Creative Commons.
Magnetic field of neutron star
Another important property of the neutron star is its extremely strong magnetic field. The shrink of the spinning objects increases not only the spinning speed but also the stellar magnetic field. The physical principle is similar to the angular momentum conservation, but it is called magnetic flux conservation; when an object shrinks in size, the magnetic field strength is inversely proportional to the square of the size. The magnetic field of neutron star is amplified during the collapse of the progenitor and reaches more than one billion Tesla, which is a billion times higher than strength of ordinary magnet in the house. Neutron star is the most strong magnetic object in the Universe.
Stellar light house: pulsar
The extremely intense magnetic field forces the radiation of the neutron star from the two poles of the magnetic field (such as North pole and South pole of the Earth). Since the focused beam is rotating with neutron star like the light house at seashore, we can only see the neutron star when the beam is pointing toward us (Figures 5 and 6). So over time, what we observe is the pulsating of the neutron star. Most of neutron stars likely undetectable because they may not emit enough radiation or just the bean never points toward us. Under some circumstances, however, the neutron stars can produce detectable pulsating radiation. Such a neutron star is called pulsar (in full pulsating radio star). Because the pulsating is so regular and the pulsars are fixed in position, the researchers have considered pulsar to navigate the travel of the space craft, just like the light house at seashore is built to guide the ship.
Scientists estimates that Swift J1818.0−1607, is only around 240 years old. So, how can we know the age of the star? Age of the star is fundamental information to classify the stars. Researchers usually use “color” of the star to estimate the age for the normal stars; younger stars appear bluer while older ones appear more red. For the pulsars, scientists use a different way to indicate the age. The arrival time between successive two pulses from the pulsars is incredibly regular and the spinning period of the pulsars can be measured very precisely. The periods of pulsars, on the other hand, are slowly and regularly increasing with time; Swift J1818.0-1607 is spinning down with a rate of around 0.003 second every year. Scientists use the ratio of the current spin period to the spin-down rate as an indication of the age, which is called “characteristic age”. The characteristic age is not exactly match with, but it can be close agreement with the true age. Swift J1818.0-1607 has the smallest characteristic age among all pulsars.
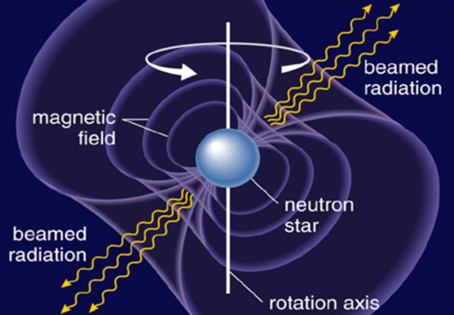
Figure 5. An artist’s impression of a radiation from the pulsar. Image credit: Copyright @2004 Pearson Education, publishing as Addison Wesley.

Figure 6 Gif animation. Light house model of the pulsar. Credit: An artist's impression of a pulsar. Image credit: Michael Kramer (JBCA, University of Manchester).
Magnetic monster: magnetar
If you spin a magnet in vacuum space, you will observe that this spinning magnet eventually stops. The spinning magnet changes the direction of the magnetic field in the space. Interesting in nature is that the changing magnetic field creates a changing electric field that creates a changing magnetic field and so on. Two fields go together and remove a spinning energy from the magnet, which causes a spinning down of the magnet. The scientists apply this idea to explain the spinning down of the pulsar. The magnetized neutron star behaves like spinning magnetic in the space, and it is not difficult to imagine that a neutron star with a higher magnetic field spins down faster. Scientists therefore use the measurement of spin-down rate to indicate the magnetic field strength of the pulsar, as well as its age. Since the first pulsar in 1967, about 3000 pulsars have been discovered and typical magnetic field strength is of the order of 0.1-1 billion Tesla.
A surprising of Swift J1818.0-1607 is that its magnetic field strength is 1000 times stronger than the typical value (Figure 7). Researchers name such a neutron star with the ultra-strong magnetic field as “magnetar” (a contraction of magnetic star). The magnetar is a rare type of the neutron stars and only about 30 magnetars have been confirmed so far. The Swift J1818.0-1607 is now known as not only the youngest, but also the fastest spinning magnetar.
The powerful magnetic field behaves like stretched rubber band and exerts a tension force on the magnetar. Over time, this stress accumulates on the surface of magnetar, and eventually crucks the surface. This leads to the star quake, akin to earth quake, and causes a violent gamma-ray outburst (Figure 8). On March 12th, 2020, Swift caught the outburst from Swift J1818.0-1607.

Figure 7 Magnetic field and characteristic age of the pulsars (black circles) and magnetars (blue circles). Swift J1818.0-1607 is the youngest among known neutron stars. Image credit: Jumpei Takata (Huazhong University of Science and Technology).
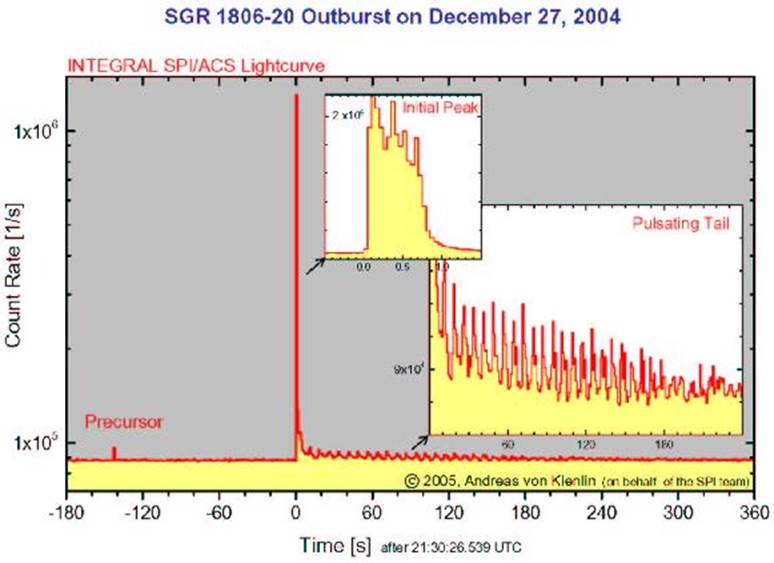
Figure 8 Gamma-ray outburst of magnetar SGR 1806-20. Image credit: A. von Kienlin, MPE.
Mystery
Because of the ultra-strong magnetic field, Swift J1818.0-1607 is classified as the magnetar. Unlike the typical magnetars, which are not seen in radio wave, Swift J1818.0-1607 however is producing pulsating radio signal and it behaves like a typical pulsar. Since the discovery of the first magnetar in 1970s[6], researchers have considered typical pulsars and magnetars are different population in neutron stars. The discovery of the radio emitting magnetar Swift J1818.0-1607 arises new question about the connection between the magnetar and typical pulsar.
The supernova explosion heats up the surface of the neutron star to very hot (about 10 million degrees). Then the neutron star is gradually cooled down by radiating X-rays. Researchers measured the cooling X-ray emission from several pulsars and magnetars, and they expected a signature of the cooling emission of Swift J1818.0-1607. However, the data of the observation does not indicate the existence of cooling emission, but indicates that the surface of Swift J1818.0-1607 is likely much cooler than the temperature expected. So, it seems the non-detection of the cooling emission is contradicting with the characteristic age of Swift J1818.0-1607. Scientists therefore still not be sure how to form the Swift J1818.0-1607 and its true age.
Hunting supernova remnant
The characteristic age determined by the spin-down rate indicates that Swift J1818.0-1607 is the youngest among known pulsars. Researchers want to detect another evidence that Swift J1818.0-1607 is truly the youngest with an age of about 240 years. Most firm estimation of the true age is the confirmation of the associated supernova remnant. Supernova explosion blows off most matter of the stars. The ejected debris coast the interstellar space with a very high speed (only about 1 second is required to go around the Earth) and can be observed as expanding nebula, which is called supernova remnant. A pulsar or magnetar is frequently detected at the center of the supernova remnant. Most famous supernova remnant is called Crab nebula. The supernova remnant of the Crab nebula was observed at 1054 on the Earth and 33-millisecond pulsar exists at the center of the Crab nebular.
Hunting the supernova remnant associated Swift J1818.0-1607 has just begun. In the next few years, deep surveys will reveal the nature of the supernova remnant and its true age of Swift J1818.0-1607.
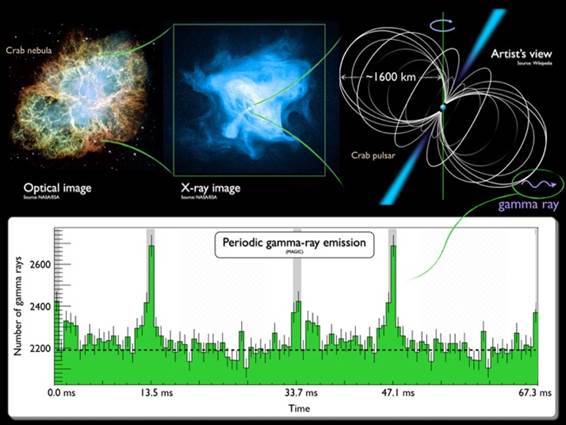
Figure 9 Supernova remnant of Crab nebula. Top left and middle panels show the image of the Crab nebular taken by the optical and X-ray telescopes. A pulsar is located at the center of the nebula. The size of the nebular is much greater than the size of the pulsar. Image credit: NASA, ESA, J. Hester, A. Loll, CXC, SAO, F. Seward et al., MAGIC Collaboration
References:
[1] Esposito, P. et al. 2020, The Astrophysical Journal Letters, Volume 896, Issue 2
[2] Evans, P. A. et al. 2020, The Gamma-ray Coordinates Network circular 27373
[3] Enoto, T. et al. 2020, The Astronomer's Telegram, No. 13551
[4] Lower, M.E. et al. 2020, The Astrophysical Journal Letters, Volume 896, Issue 2
[5] Hewish, A., Bell, S.J. et al. 1968, Nature, Volume 217, Issue 5130
[6] Mazets, E. P. and Golenetskii, S.V., 1981, Astrophysics and Space Science, Volume 75, Issue 1
作者简介
Jumpei Takata,华中科技大学天文系教授,主要研究领域伽玛射线脉冲星。
版权声明:原创论文,转载需注明出处。
编校:雷卫华,邹远川 许文龙
文章编号:华中大天文200717A